Variable Fertilizer Application Improves Profits and Conservation
Chapter 6 – Fertility, No. 13, Spring 1989
Roger Veseth
Natural soil variability is a fact of life that producers must deal with on much of our Northwest cropland, Yield potentials, fertilizer requirements and management problems often vary greatly within fields whose margins are delineated largely on survey boundaries rather than landscape position and production potential.
In the central Palouse region of the Inland Northwest, for example, yields of winter wheat, across the rolling field landscape, can often vary from 30 to 100 bushels per acre when the field average is 75 bushels per acre. When uniformly applying nitrogen (N) fertilizer for a 75 bushels per acre yield in such a field, highly productive areas would be under-fertilized, reducing potential yields and profits. Conversely, low yield potential areas would be over fertilized, reducing profits from unnecessary fertilizer costs .
The over-fertilization also increases the potential for excess protein content in soft white wheat. In addition, excess N fertilizer could become a source of contamination for surface and ground water.
Management Strategies
Many Northwest cropland fields have contrasting areas with distinctive soil properties, production potentials and limitations. If these areas can be delineated for management purposes, then knowledge of these distinct conditions can be used to develop special production strategies. One common management option is the adjustment of fertilizer rates and blends for the particular soil, in order to optimize potential yield with available water and to improve fertilizer use efficiency. Tillage practices and other cultural management options could also be adjusted for the particular soil and crop production conditions.
Identifying field soil variability and developing crop management strategies for variable soil conditions are the focus of some of the research underway by Dave Mulla, Washington State University soil scientist and STEEP researcher at Pullman. STEEP (Solutions to Environmental and Economic Problems) is a cooperative conservation farming research effort involving more than 100 scientists in Oregon, Idaho and Washington. Mulls proposes that soil, water and fertilizer resources can be used most efficiently with a variable fertilizer management strategy matched to spatially variable crop yield potentials within the field.
In Fall 1987, Mulls established two field studies to evaluate the possibility of replacing the traditional uniform fertilizer application with variable rates and blends of fertilizer according to patterns in soil test results and production potentials. They were conducted in cooperation with two Cenex agronomists, Max Hammond at Ephrata, WA, and Jim Benson at Moscow, ID.
Field Studies
Location and Research Focus
The field studies were conducted near St. John and Colfax, WA. The St. John site was a sharply rolling landscape with exposed subsoil or shallow topsoil on the eroded hilltops and ridges, and thick topsoil on the lower slopes and bottomland. . . a common situation in much of the Palouse region. Soil samples at the St. John site were taken every 50 feet, on four east-west oriented parallel transects 2,000 feet long and 400 feet apart (160 samples). The soil cores were taken to a depth of 6 feet and divided into l-foot increments for analyses. Water and nitrate-nitrogen (NOS-N) content were measured on each foot increment. Analyses for phosphorus (P), ammonium-nitrogen (NH1-N), pH and organic matter (OM) content were made on the top 2 feet.
In contrast to the sharply rolling St. John site, the Colfax site was on a broad gentle slope with no distinct visual differences in soil productivity. The research design was similar to the one in St. John except that there were only three parallel transects.
The St. John site covers about 59 acres in size and the Colfax site about 40 acres. Both were in conventional summer fallow after winter wheat, The uniform fertilizer applications, used by the farmer, and the variable fertilizer applications were made in adjacent strips along the sampling transects. A commercial fertilizer applicator was used for fertilizer injection at a depth of 5 inches. The entire St. John site also received an aerial application of 30 pounds N per acre in the spring. Stephens winter wheat was planted at both sites in early October.
Variable Fertilizer Application Strategy
As in any sound fertility program, the variable fertilizer application rates for each soil sample site on the transects were based on three factors affecting production potential: (1) estimated yield potential based on projected available water, (2) soil test nutrient levels and (3) projected N mineralization (release) in the soil during the growing season.
Yield potential was determined from an equation in the WSU Winter Wheat Fertilizer Guide FG-34: potential winter wheat yield = 7 bushels per acre x (inches of growing season precipitation expected + inches stored soil water to a 6 foot depth – 4 inches). Four inches of available water is subtracted in the equation since this amount is needed to grow the plant to the reproductive stage, after which additional available water will increase potential yield.
Mulla assumed an expected growing season precipitation of 8 inches at both sites from February to July. Actual measured precipitation at the sites during that period was 7.8 inches at St. John and 8.2 inches at Colfax. Expected increases in soil water storage overwinter, between the September 1987 soil water sampling and the February growing season precipitation, were also included in the available water estimates for predicting yield potentials. A uniform storage of precipitation in the soil across the landscape was assumed in estimating total available soil water for yield potential. Mulls points out, however, that surface runoff probably redistributed some of the precipitation across the landscape, Available soil water content in September, to 6 feet depth, was strongly influenced by landscape position (Fig. 1).
The estimated potential yield at each sampling site on the transects is illustrated in Fig. 2. As would be expected, the potential yield correlated quite closely to topographic position, as did available water (Fig, 1). The lowest yield potentials are typically on the hilltops and highest yield potentials on the lower slopes and bottomland. Available soil nutrient levels for each site on the transect were determined from the soil test analyses, Topographic position significantly influenced the level of available P (Fig. 3) and NO3-N (Fig. 4) in the soil.
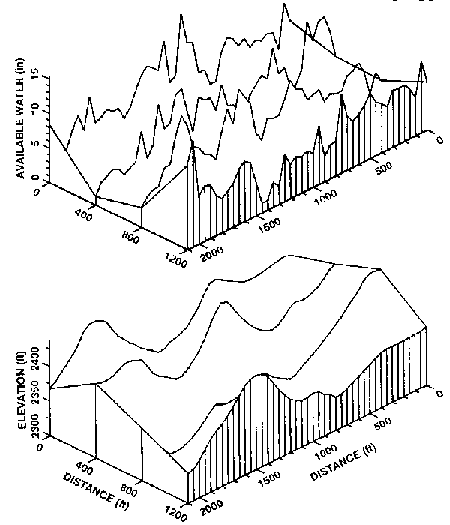
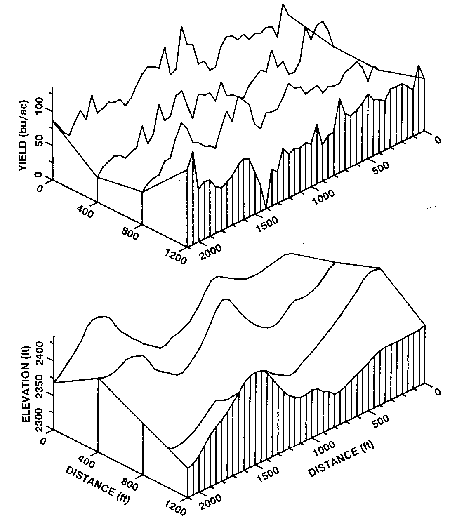
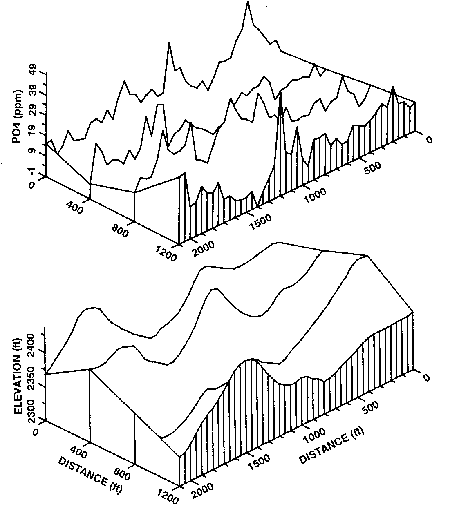
Reduced P availability on the hilltops and steep upper slopes was associated with loss of the fertile topsoil and exposure of the less fertile, less productive subsoils. These areas also typically had the lowest N03-N content to 6 feet. High N03-N content was found on the bottomland, associated with the highest OM and available water contents, and corresponding soil biological activity.
In addition to the N03-N and NH~-N soil tests, the estimated mineralization of N from organic matter decomposition during the crop year must be included in the crop N fertility budget. It was estimated that 50 pounds N per acre would be mineralized from soil containing 3 percent OM; 40 pounds N per acre from 2 percent OM; and 30 pounds N per acre from 1 percent OM. Soil OM content also correlated quite closely to topographic position at the St. John site (Fig. 5). The lowest OM content was generally on the hilltops and highest was on the lower slopes and bottomland.
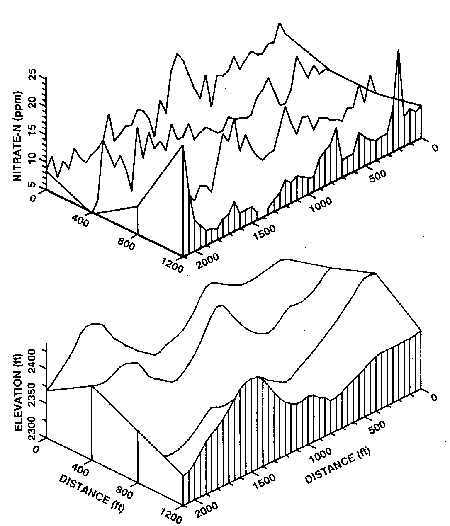
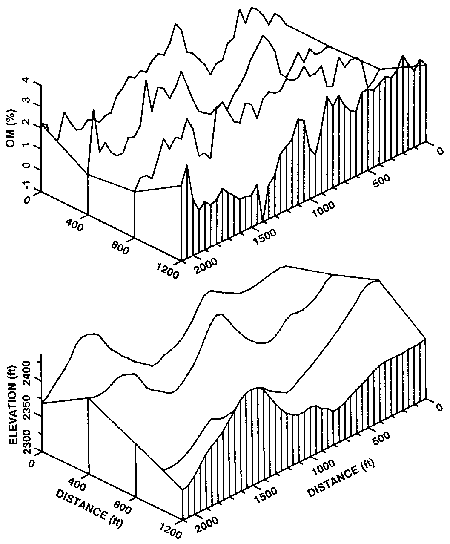
Fertilizer application rates were then determined from the WSU Winter Wheat Fertilizer Guide for each sample site on the transects. The variability of P and N fertilizer rates across the transect topography at the St. John site are illustrated in Figs. 6 and 7.
Fertilizer blends of N and P were then established for each of the 160 sample sites on the transects at the St. John site and 120 sample sites at Colfax. Originally, there were nine combinations of low, medium and high rates of P and N fertilizer at the St John site. For a more manageable fertilizer application program, Mulls divided the application rates into three management units (Table 1). Three variable fertilizer management units at the Colfax site included only N fertilizer.
The uniform fertilizer application used by the farmer at the St. John site included 65 pounds N per acre and 20 pounds P20~ per acre, The farmer originally planned to use 75 to 80 pounds N per acre and no P, but made the adjustments in his fertilizer plans after seeing the soil test results. All the variable and uniform application treatments received 15 pounds sulfur (S) per acre in the fall and a spring aerial application of 30 pounds N per acre.
Table 1. Variable fertilizer management units for the transect sample sites and representative percentage of the transect lengths at the research sites near St. John and Colfax,WA; 1987 (Mulla, WSU).
Management Units for N and P Rate Fertilizer Blends | Portion of Transect Length (%) | Fertilizer Rate (lb/acre) |
|
---|---|---|---|
N | P(P2O5) | ||
St. John Site | |||
Low N, low P | 38 | 20 | 12 |
Medium/high N, low P | 31 | 80 | 20 |
Low N, high P^1 | 31 | 20 | 40 |
Colfax Site | |||
No fertilizer | 48 | 0 | 0 |
Low N | 29 | 25 | 0 |
Medium N | 23 | 50 | 0 |
*This unit includes six combinations of low to high N and medium to high P fertilizer rates which represented small portions of the total transect length. |
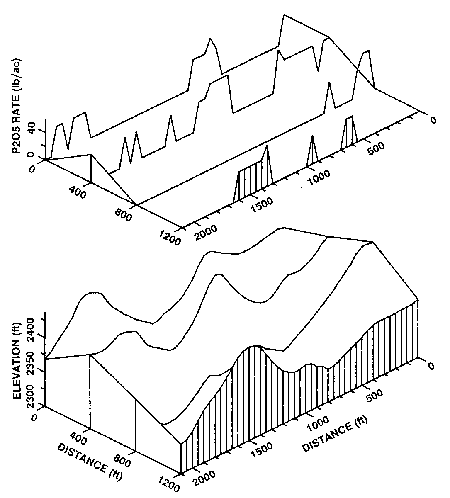
The uniform fertilizer application used by the farmer at the Colfax site was 90 pounds N per acre and 15 pounds S per acre, No S was applied in the variable fertilizer treatments. No P was applied in either the variable or uniform fertilizer applications.
Results
Mean yields and test weights with the variable fertilizer application rates were only slightly greater than those with the uniform rates at both study sites (Table 2). The important advantage was that a lower fertilizer input was required in the variable fertilizer strategy.
The value of soil testing was particularly dramatic at the relatively uniform Colfax site. Because of the residual soil N level, detected only by soil tests, there was nearly a $14 per acre reduction in fertilizer N expense compared to the uniform application by the farmer. Nearly one-half of the field (Table 1) required no fertilizer and about another one-third required only 25 pound N per acre, in contrast to the 90 pounds N per acre “traditional” uniform rate.
Table 2. Mean winter wheat yield and test weight, fertilizer cost and net benefit of a variable fertilizer application compared to a uniform fertilizer application in field studies near St, John and Colfax, WA, 1988 (Mulla, WSU).
Fertilizer Application | Yield* (bu/acre) | Test Weight (lb/bu) | Fertilizer Cost (per acre) | Net Benefit of Variable Rate** |
---|---|---|---|---|
St. John Site | ||||
Variable | 63.5 | 61.6 | $18.95 | $11.20 |
Uniform | 61.7 | 61.1 | $22.05 | - |
Colfax Site | ||||
Variable | 57.7 | 59.7 | $2.58 | $31.77 |
Uniform | 53.7 | 58.5 | $16.35 | - |
*Adjusted to 60 lb/bu test wt. **Based on $4.50/bu wheat and reduced fertilizer costs. |
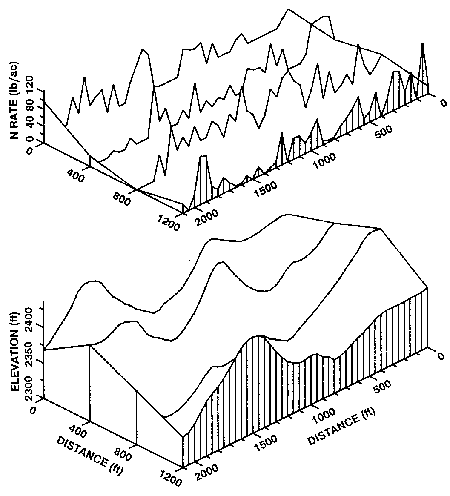
Some additional soil testing expense would probably occur in delineating field management units and determining baseline soil test properties. In an actual farm management situation, however, the number of samples would be only a small fraction of those used in this research study. Once the baseline data are established on those management units, only yearly sampling for soil N would be needed. Routine sampling for other nutrients and soil properties every few years would be advisable to monitor changes.
Management Implications
Soil Testing Pays
Mulls points out that one of the most obvious management implications of the study is that ”soil testing pays.” He also stresses that when soil sampling, it is important to make separate composite soil samples for each identifiable soil management unit within the field.
Relationship to Landscape Position
The study has provided an opportunity to assess the effect of landscape position and associated soil properties on production potential and management choices. Mulls found that soil OM, P and N content, soil water content, yield potential and required fertilizer application to achieve potential yields generally correlated closely with landscape position and soil color. At the St. John site, for example, the eroded, light-colored hilltops and upper slopes typically were dryer and had a lower OM content, lower P and N soil tests, and lower potential yield than the dark colored, non-eroded soils on the lower slopes and bottomlands. These eroded areas generally required higher P and lower N fertilizer applications to attain the yield potential with available water. The combination of landscape position, soil color, soil test results and other considerations should help producers delineate fertility management units within fields.
Increased Profits from Conservation Practices
This approach also lends itself to the possibility of subdividing fields into more homogeneous units for other management decisions in addition to fertility. It has special application to conservation practices such as divided slope and contour strip farming on sloping cropland. In addition to the advantage of these practices for reducing runoff and soil erosion potential, the practices also allow adjustments for improving fertilizer and tillage management on different soil areas. For example, a contour strip which includes the eroded hilltops and ridges would require a different fertilizer blend than contour strips with the more-productive lower slopes and bottomland. In addition, differences in crop residue production allow different options in tillage systems. Lower residue production on the upper contour strips facilitates the adoption of reduced tillage and no-till practices. High residue production and less erosion potential on the lower slopes and bottomland would allow more intensive tillage operations.
The use of conservation tillage practices and contour strips or divided slopes would also tend to even-out crop yield potential with available water across a rolling landscape. These practices help hold precipitation where it falls, increasing soil water storage and yield potential. The greatest benefit of these conservation practices for holding precipitation in place is on the dryer, runoff-prone areas of the field which typically produce less crop residue for reducing runoff and evaporation.
STEEP research has demonstrated that conservation tillage practices, which maintain about 30 to 50 percent surface cover with crop residue, can reduce runoff and evaporation by 60 percent or more when compared to residue-free conventional tillage. This has commonly resulted in about 2 inches more available soil water at research sites in the 16-to 20-inch annual precipitation areas of the Inland Northwest. With increased soil water storage on the runoff-prone hilltops and steeper slopes, variable fertilizer rates could then be adjusted to take advantage of the improved yield potentials.