Soil Water Use and Growth of Russian Thistle After Wheat Harvest
Chapter 5 – Weed Control Strategies, December 1999
Author: William Schillinger, research agronomist, Department of Crop and Soil Sciences, Washington State University, Lind; Frank Young, research agronomist, USDA-Agricultural Research Service, Pullman; Harry Schafer, research technician, Department of Crop and Soil Sciences, WSU, Lind; Larry McGrew, research technician, USDA-Agricultural Research Service, Pullman.
Abstract
Russian thistle (Salsola iberica Sennen and Pau) is a major broadleaf weed in dryland crops (less than 12 inch annual precipitation) in the Pacific Northwest of the USA. Russian thistle frequently infests wheat (Triticum aestivum L.) and other spring-sown crops, especially during drought. Quantitative information on water use, biomass accumulation, and seed production of Russian thistle after wheat harvest is lacking. In a 2-yr field study at Lind, Washington, Russian thistle plants were allowed to grow yearly in spring wheat in a grid pattern without competition from other weeds. Individual Russian thistle plants used 20 gallons of soil water while growing with the crop. From wheat harvest in early August until killing frost in late October, each Russian thistle used an additional 27 gallons of soil water. Water use occurred within a 5-ft radius of the Russian thistle. Spring wheat competed with Russian thistle for water at shallow soil depths; most water use by Russian thistle was from deeper than 1.0 m. Russian thistle dry weight increased from 0.4 to 2.8 lbs per plant between grain harvest and killing frost. Russian thistle seeds were either not produced or germinable until mid-September. By late October, individual plants had produced 67,000 and 25,000 seeds in 1996 and 1997, respectively. Water recharge into the soil was reduced near Russian thistle after a winter of high precipitation where water infiltration occurred to depths greater than 3 ft, but was not affected the next year when winter precipitation was close to the long-term average. In low crop residue situations, rapid post-harvest growth by Russian thistle (before seed production) provides valuable surface cover for erosion control, but with the prospect that soil water may be reduced for the subsequent crop.
Introduction
Russian thistle is a summer annual weed which has long plagued crop production in arid and semiarid regions of the western United States and Canada (Dewey, 1893). It is the dominant broadleaf weed in the 3.5 million acre dryland (6 to 12 inch annual precipitation) crop production region of the inland Pacific Northwest. The traditional cropping system is winter wheat with tillage-intensive fallow in alternate years. Drawbacks to the wheat-fallow system include: 1) recurrent wind and water erosion (Papendick, 1998), 2) decline in soil organic matter due to carbon loss by biological oxidation exceeding carbon input from residue (Rasmussen and Parton, 1994), and 3) inefficient storage of precipitation in the soil during the spring-summer of the fallow cycle and the fall-winter of the crop cycle (Ramig and Ekin, 1991). Consequently, many growers in this dryland region are increasing the intensity of spring cereal cropping (i.e., decreasing the frequency of fallow) and reducing or eliminating tillage.
Russian thistle presents a formidable obstacle to successful dryland spring cropping. Spring wheat and spring barley (Hordeum vulgare L.) have less early growth and slower canopy closure compared with winter wheat which grows vigorously in early spring. Infestation is most severe when crop competition is reduced by poor stands, drought, inadequate fertility, and late growth. Russian thistle seedlings first emerge in March or April, flower in June, and produce seed beginning in August. Grain yield of spring cereals may be reduced by 50% or more in severe infestations (Young, 1988). Grain quality is also diminished when green Russian thistle biomass and seed contaminate it and increase moisture (Holm et al., 1997). Russian thistle also infests broadleaf crops which are needed to diversify the present cereal-only cropping system. Herbicides for Russian thistle control in broadleaf crops are either not labeled, have rotational restrictions, or require incorporation and, therefore, are not compatible with no-till systems.
The root system of Russian thistle can extend 6 ft deep and 16 ft in diameter (Holm et al., 1997). Russian thistle is C4 plant with high water use efficiency (Dwyer and Wolde-Yohannis, 1972). When not controlled, mature plants dislodged from the soil by wind, decay, or tillage can scatter seed over several miles (Stallings et al., 1995). Growers typically either till with V-shaped sweeps or use herbicides for post-harvest Russian thistle control before the onset of seed production. Sweep tillage cuts the Russian thistle roots, but also buries some crop residue, and severed Russian thistle (tumble weeds) blow away. This is a concern in low-residue situations where Russian thistle stands have often produced more dry biomass than the crop by time of grain harvest in late July-early August (Schillinger et al., 1999). Therefore, dead Russian thistle may be an important source of surface cover for erosion control, especially if beginning a 13-mo-long fallow cycle. In addition, increased quantities of residue enhance over-winter water storage (Papendick and Miller, 1977). When post-harvest control is with herbicides, Russian thistle generally remain anchored in the soil or trapped by standing stubble or snow.
For growers to convert from traditional winter wheat-fallow to more intensive farming involving spring crops, further understanding of the ecology and management of Russian thistle is needed. The objective of this study was to determine the effects of individual Russian thistle plants when allowed to grow uncontrolled in spring wheat and after crop harvest on: i) the extent and pattern of soil water extraction and impact on over-winter soil water content, ii) post-harvest Russian thistle dry biomass accumulation and, iii) timing and magnitude of Russian thistle seed production.
Methods and Materials
Overview and Field Layout – A 2-year field study was conducted from February 1996 to March 1998 at the Washington State University Dryland Research Station at Lind, WA. The soil is a deep (more than 6 ft to bedrock) Shano silt loam. Average annual precipitation is 9.61 inches, which is less than any other state or federal non-irrigated crop research facility in the United States.
In late February of 1996 and 1997, 12 oz. glyphosate (Roundup) was applied (in stubble) to control winter annual grass weeds and volunteer wheat before planting spring wheat. Plots were tilled with a duck-foot cultivator with an attached 4-bar harrow, and fertilized with a Solution 32 blend of 50 lbs N, 16 lbs P, and 10 lbs S per acre. In mid-March of each year, hard red spring wheat (cv. Butte 86) was sown at 70 lbs per acre with a double-disc drill in 6-inch-wide rows. When wheat tillered in mid-to-late April, paper cups were placed over 100 juvenile Russian thistle in a 20 x 20-ft grid (one plant every 400 square feet). Unprotected Russian thistle were killed with 12 oz./acre of bromoxynil. Russian thistle that germinated later were removed by hand. Wheat was harvested with a combine cutting 12 inches above the ground on 1 August both years. About 20% of total Russian thistle green biomass was removed from the top of each of the 100 plants during harvest.
Soil Water Use – Within 2 days after wheat harvest, aluminum access tubes were installed at distances of 1, 2, 3, 5, and 10 feet from the base of individual Russian thistle plants. The experimental design was a randomized complete block with 6 replications (i.e., individual Russian thistle plants), with each block containing the set of 5 access tubes. The access tube located 10 ft from each plant was the control treatment from which we assumed no water extraction by Russian thistle would occur.
At about 13-day intervals from the first week of August until after killing frost in late October, soil volumetric water content was measured in 6-inch increments to a depth of 6 ft by neutron attenuation (Gardner, 1986). Overall mean water use by the six individual Russian thistle on each sampling date was determined by summing water depletion for each distance (compared with the control treatment located 10 ft from each target Russian thistle) and using conversion factors to calculate the surface area represented by the respective access tubes. Neutron access tubes located 1, 2, 3, and 5 ft from individual Russian thistle plants represented surface areas of 7 ft2, 13 ft2, 19 ft2, and 40 ft2, respectively (79 ft2 collectively). Access tubes remained in place over the winter, until soil water recharge was measured in late February or in March of the following year.
An analysis of variance (ANOVA) was conducted for soil water on every measurement date for each 6-inch depth increment as well as the entire 6 ft soil profile. Treatments were considered significantly different at P < 0.05. Treatment means for all ANOVA in this study were separated using Fisher’s protected least significant difference.
Results and Discussion
Soil Water Use – At spring wheat harvest on 1 August in 1996 and 1997, individual Russian thistle plants had already used 19 gallons or more water (Fig. 1). Spring wheat competed with Russian thistle for water at shallow soil depths, as evidenced by no differences in soil water content among access tube treatments in early August until depths of 2.5 ft in 1996 and 4 ft in 1997 (data not shown). However, Russian thistle had already depleted soil water below these depths at harvest. These data agree with scanner rhizotron root observations in a separate study at Lind in 1997 showing prolific lateral rooting of Russian thistle at soil depths of 2 ft and below (W.L. Pan, unpublished data).
Measured water extraction by Russian thistle was always greatest closest to the plant and decreased proportionate to distance from the plant on all August-to-October sampling dates during both years (data not shown). Whereas spring wheat extracts water relatively inefficiently from soil depths deeper than 4 ft, Russian thistle aggressively reduced soil water content at 1 ft from the plant to 5% by volume throughout the entire 6 ft profile in 1996 and to a depth of 4.5 ft in 1998 (data not shown). Individual Russian thistle did not extract water beyond a 5-ft radius of the base of the plant, as there were no significant water differences at any depth or on any sampling date between measurements obtained 5 ft and 10 ft from the Russian thistle plants. In both years, Russian thistle depleted soil water until killed by hard (250F) frost on 23 October in 1996 and 25 October in 1997 (Fig. 1). Individual plants had removed an average of 47 gallons of residual soil water by late October (Fig. 1), in addition to most of the August-through-October precipitation (2.4 inches in 1996 and 1.8 inches in 1997). The 47 gallons amounts to 0.9 inch of soil water from within the 79 ft2 extraction zone of each Russian thistle, or 25,000 gallons per acre if plants were uniformly spaced 10 ft apart. The loss of this quantity of soil water would reduce grain yield of a subsequent wheat crop by about 6 bushels per acre according to Leggett (1959).
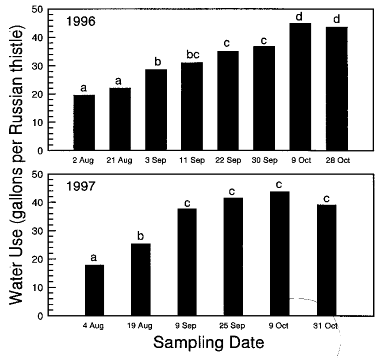
Over-Winter Soil Water Recharge – In the Pacific Northwest, soil water recharge occurs during fall and winter. Precipitation at Lind between 1 October and 1 March was 9.48 inches in 1996-1997 and 5.51 inches in 1997-1998, compared with the 80-year average of 5.28 inches. Surface soils were only briefly frozen during both winters, and water did not runoff from the site either year. After the wet 1996-1997 winter, partial soil water recharge had occurred to a depth of at least 6 ft and soil water content in the control treatment 10 ft from the Russian thistle plant was significantly higher than other treatments (data not shown).
Precipitation between 1 October-1 March was close to the long-term average in 1997-1998 and soil water recharge was much less than in the previous wet winter (data not shown). Uniform, but meager, water recharge occurred in all treatments in the top 2.5 ft of soil, but decreased sharply below this depth. There were no significant differences at any depth or in total profile water among the treatments in late February 1998. Because Russian thistle extracts water from below the rooting depth of spring wheat, these data suggest that soil water storage may not be adversely affected by Russian thistle during “average” years when over-winter recharge occurs to only 3 ft or less.
Dry Biomass Accumulation, Seed Production, and Germination – Dry biomass of individual Russian thistle plants averaged over 2 years was 0.4 lbs just after spring wheat harvest but increased rapidly thereafter (Fig. 2). In 1996, dry biomass accumulation peaked by 22 September, but continued steadily until late October in 1997. During both years, final dry weight exceeded 2.7 lbs per plant (Fig 2).
Russian thistle did not produce seed until mid-to-late September in 1996, whereas plants had already produced some seed at the time of spring wheat harvest in early August in 1997 (Table 1). Total Russian thistle seed production per plant in 1996 (58,350) and 1997 (25,070) were much greater than that reported following spring wheat at Lind (17,400) by Young (1986). In that (1986) study, a frost killed the Russian thistle plants on 23 September whereas the growing period extended until the end of October in both 1996 and 1997. Russian thistle is an indeterminate plant which will continue to grow and produce seed until the temperature drops to about 250F for one night or just below 320F for several successive nights (Young et al., 1995). Although Russian thistle produced seed by early August in 1997, seeds were not germinable until 9 September (Table 1). Germination increased from 23% for seed collected on 9 September to 48% for seed harvest on 31 October. Although the germination data were only obtained in one year, these results agree with other studies in the Pacific Northwest (Young and Whitesides, 1987) and other regions of the world (Holm et al., 1997; Young and Evans, 1972) that germination increases with after- ripening in the field.
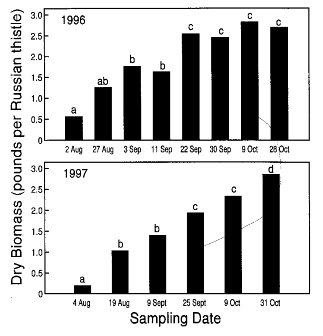
Table 1.
1996 | 1996 | 1997 | 1997 | 1997 |
---|---|---|---|---|
Date | Seed Production (# of plants) | Date | Seed Production (# of plants) | Germ. (%) |
2 August | 0 a | 4 August | 100 a | 0 a |
21 August | 0 a | 19 August | 190 a | 0 a |
3 September | 0 a | 9 September | 500 b | 23 b |
11 September | 0 a | 25 September | 7,600 bc | 30 bc |
22 September | 4,530 b | 9 October | 10,830 c | 40 c |
30 September | 10,290 b | 31 October | 25,070 d | 48 d |
9 October | 67,400 c | |||
28 October | 58,350 c | |||
† Within column means followed by the same letter are not significantly different at the 0.05 probability level. |
Summary and Management Recommendations
Water is the most limiting factor in dryland crop production regions where Russian thistle is the dominant broadleaf weed. Russian thistle aggressively extracted soil water, beyond the available range of spring wheat as well as from deeper soil depths than spring wheat, until the weed was finally killed by frost. Russian thistle plants produced an average 46,000 seeds between early August and late October, but seeds were either not produced or germinable in appreciable quantities until mid-September.
A management option for erosion control in extreme low-crop-residue situations is to allow Russian thistles to grow for a period of time after wheat harvest prior to germinable seed production. In this study, an average of 1.6 lbs dry biomass per Russian thistle (about 885 lbs per acre) was produced during this 5 to 7 week post-harvest window. However, Russian thistle used 0.35 inch of soil water to produce this biomass, which could reduce the subsequent wheat yield by about 2.5 bushels per acre.
In the wheat-summer fallow rotation, we feel the best post-harvest management strategy in low-crop-residue situations with heavy Russian thistle infestation is to apply a fast-acting herbicide. Herbicide should be applied 10 to 14 days after wheat harvest when Russian thistle begins rapid regrowth (Young, 1986). This will halt soil water use and seed production and dead Russian thistles will be kept in place as source of residue for erosion control. In addition, over-winter soil water storage will likely be augmented due to the additional soil surface cover. Post-harvest sweep tillage to sever the roots of Russian thistle is not advised when residue is lacking as dislodged plants will be wind-blown from the field. In the spring of the fallow cycle, primary tillage with non-inversion wide-blade sweeps may be followed by 2 or 3 secondary tillage operations with rodweeders in late spring and summer to control Russian thistles and other weeds. This method has proven to consistently retain more than the minimum 350 lbs per acre surface cover (Schillinger et al., 1999) required for highly erodible soils.
Post-harvest control of Russian thistle before germinable seed production is especially important when the ensuing crop will be spring wheat. Wind erosion is less a factor when spring cropping compared with summer fallow, thus either herbicide or sweep tillage are acceptable post-harvest Russian thistle management options. Spring wheat sown i) early and with minimum soil covering the seed for fast emergence and, ii) on narrow (6 to 9 inch) row spacing, will increase the crop’s competitiveness with Russian thistle. On-going research to suppress Russian thistle by spring wheat varieties with rapid and prostrate early growth habit, and effective in-crop herbicides with short-term residual activity, will help make spring cropping an increasingly viable option for inland Pacific Northwest drylands.
References
Dewey, L.H. 1893. The Russian thistle and other troublesome weeds in the wheat region of Minnesota and North and South Dakota. USDA Farmers’ Bull. 10. 16 p.
Dwyer, D.D. and K. Wolde-Yohannis. 1972. Germination, emergence, water use, and production of Russian thistle. Agron. J. 64:52-55.
Gardner, W.H. 1986. Water content. p.493-544. In A. Klute (ed.) Methods of soil analysis. Part 1. 2nd ed. Agron. Monogr. 9. ASA and SSSA, Madison, WI.
Holm, L., J. Doll, E. Holm, J. Pancho, and J. Herberger. 1997. Salsola kali L. p. 708-721. In World weeds: Natural histories and distribution. John Wiley & Sons, New York.
Leggett, G.E. 1959. Relationships between wheat yield, available moisture and available nitrogen in eastern Washington dry land areas. Wash. Agr. Exp. Sta. Bull. 609:1-16.
Papendick, R.I. 1998. Farming with the wind: Best management practices for controlling wind erosion and air quality on Columbia Plateau croplands. Washington State Univ. College of Agric. and Home Econ. Misc. Pub. no. MISC0208.
Papendick, R.I., and D.E. Miller. 1977. Conservation tillage in the Pacific Northwest. J. Soil Water Cons. 32:49-56.
Ramig, R.E., and L.G. Ekin. 1991. When do we store water with fallow? Columbia Basin Agric. Res., Oregon Agric. Exp. Stn. Rpt. 860:56-60.
Rasmussen, P.E., and W.J. Parton. 1994. Long-term effects of residue management in wheat-fallow: I. Inputs, yield, and soil organic matter. Soil Sci. Soc. Am. J. 58:523-530.
Schillinger, W.F., R.I. Papendick, R.J. Veseth, and F.L. Young 1999. Russian thistle skeletons provide residue in wheat-fallow cropping systems. J. Soil Water Cons. 54(2):506-509.
Stallings, G.P., D.C. Thill, C.A. Mallory-Smith, and L.W. Lass. 1995. Plant movement and seed dispersal of Russian thistle (Salsola iberica). Weed Sci. 43:63-69.
Wallace, A., W.A. Rhods, and E.F. Frolich. 1968. Germination behavior of Salsola as influenced by temperature, moisture, depth of planting, and gamma irradiation. Agron. J. 60:76-78.
Young, J.A. and R.A. Evans. 1972. Germination and establishment of Salsola in relation to seedbed environment. I. Temperature, after ripening, moisture relations of Salsola seeds as determined by laboratory studies. Agron. J. 64:214-218.
Young, F.L. 1986. Russian thistle (Salsola iberica) growth and development in wheat (Triticum aestivum). Weed Sci. 34:901-905.
Young, F. L. 1988. Effect of Russian thistle (Salsola iberica) interference on spring wheat (Triticum aestivum). Weed Sci. 36:594-598.
Young, F., R. Veseth, D. Thill, W. Schillinger, and D. Ball. 1995. Managing Russian thistle under conservation tillage in crop-fallow rotations. Pacific Northwest Ext. Pub. 492. Univ. of Idaho, Oregon State Univ., and Washington State Univ.
Young F.L. and R.E. Whitesides. 1987. Efficacy of postharvest herbicides on Russian thistle (Salsola iberica) control and seed germination. Weed Sci. 35:554-559.
Pacific Northwest Conservation Tillage Handbook Series publications are jointly produced by University of Idaho Cooperative Extension System, Oregon State University Extension Service and Washington State University Cooperative Extension. Similar crops, climate, and topography create a natural geographic unit that crosses state lines in this region. Joint writing, editing, and production prevent duplication of effort, broaden the availability of faculty, and substantially reduce costs for the participating states.
Cooperative Extension programs and policies comply with federal and state laws and regulations on nondiscrimination regarding race, color, gender, national origin, religion, age, disability, and sexual orientation. The University of Idaho Cooperative Extension System, Oregon State University Extension Service and Washington State University Cooperative Extension are Equal Opportunity Employers.