Managing Variable Soils
Chapter 6 – Fertility, No. 6, Fall 1986
Roger Veseth
The variability of soil properties across farm landscapes impacts land management for crop production potential as well as soil and water conservation, Only a limited amount of data has been collected in the Northwest on variability of soil properties on a field basis and little methodology for integrating the data into management systems. To develop a better understanding of soil variability for improved land management, a new STEEP research project was established in 1985 by Dave Mulla, Washington State University soil scientist at Pullman. Cooperators on the project include Don McCOO1, USDA-ARS agricultural engineer, WSU soil scientists Alan Bussaca and Bruce Frazier, and other STEEP researchers.
Research Objectives
There are three main objectives of Mulla’s STEEP research effort.
- To develop a limited data base on soil properties affecting soil erosion potential and crop production. . . For this purpose, data are being collected from transects across four Palouse area watersheds, each about 2,000 acres in size. The eastern Washington watersheds near Lacrosse, Colfax, Thornton and Garfield have distinctly different landforms and degrees of soil variability.
- To develop methodology for analysis of spatial variability of selected soil properties within each watershed and their correlation with topographic factors as well as with other soil properties. . . To achieve this, computer models are being developed to predict soil properties for soil series, slope aspect, slope steepness and position on the landscape.
- To develop models to simulate runoff and erosion based on the variable soil, hydrologic and topographic parameters of the watershed. . . Presently, the Universal Soil Loss Equation (USLE) cannot take soil variability into account in predicting soil losses.
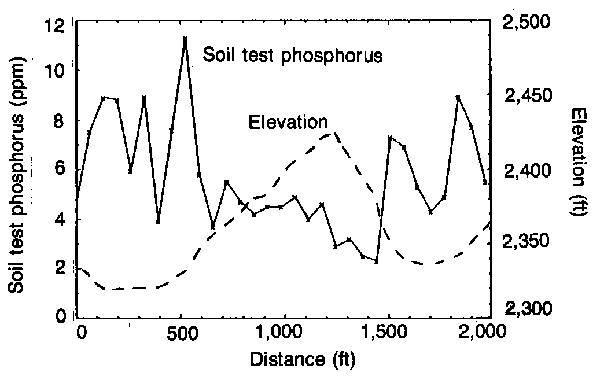
Example of the Research Impact
The data collected from this project can be important from a management standpoint. For example, research results on the variability of soil test phosphorus (Fig. 1) show the relationship between elevation and soil test phosphorus levels across a 2,000-foot (west-east) transect in a field in the Thornton area watershed.
The hilltop area with shallow topsoil has a relatively low but uniform soil test phosphorus level. In contrast, lower slope positions have soil test phosphorus levels that are generally higher and more variable. This points out the need for different phosphorus fertilizer rates on the hills compared to deeper soils on the lower slopes. Mulla’s research indicates that soil test phosphorus levels have landscape-related trends which appear to follow soil organic matter content. Correlations similar to this with other plant nutrients and soil properties could improve management accuracy without additional sampling and analyses. In Columbia Basin studies, Mulls has found that soil test phosphorus and soil test potassium have similar landscape related trends.
Mulla’s computer modeling of soil test phosphorus on about 6 acres of an irrigated field in the Columbia Basin provide an interesting picture of soil test phosphorus levels and fertilizer needs. Soil test phosphorus was measured on soil samples obtained from a 100-foot sampling grid (Fig, 2). A 5 ppm contour interval is also shown. A computer interpolated map of the field (Fig. 3) shows soil test phosphorus at 1 ppm contour intervals. Suggested phosphorus fertilizer rates (as PzO~) for each 5 ppm contour interval (Fig. 3) are shown in Fig. 4. With these computer developed maps, productivity could be increased through more efficient use of fertilizer and increased yield potential for the field.
A common question of growers and the agricultural service industry is “What intensity of soil sampling is required for accurate fertilizer application on fields with variable soils? “. Mulla’s computer analysis efforts may also help address this concern. Using the same field area (Figs. 2-4) as an example, Mulls developed 3-dimensional computer maps of soil test phosphorus at three sampling grid sizes. Soil sampling grids of 100 feet (4.4 samples/acre) and 200 feet (1, 1 samples/acre) adequately located areas of significantly different soil test phosphorus levels to allow for accurate phosphorus fertilizer application (Fig. 5). At a sampling grid of 400 feet (1 sample/2 acres), however, accurate illustration of the soil test phosphorus across this field was not possible. This does not mean that all soil sampling should be on a grid of 200 feet or less. But, it does help show the potential value of Mulla’s methodology for evaluating the variability of soil properties and its application for improved on-farm management systems.There is an increasing need in today’s farm economy for refinement of land management systems to reduce production costs while maintaining or increasing production potential.
With better understanding of the variability of soil properties, the relationships between soil properties and soil property-topographic relationships, growers will be in better positions to increase production efficiency.
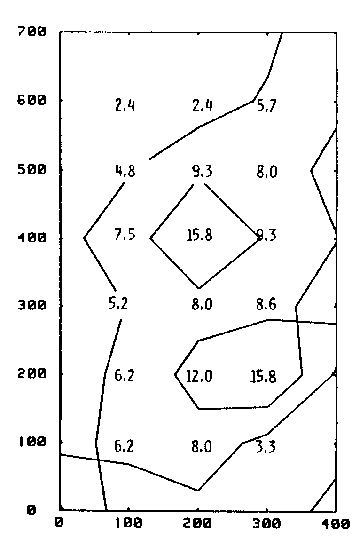
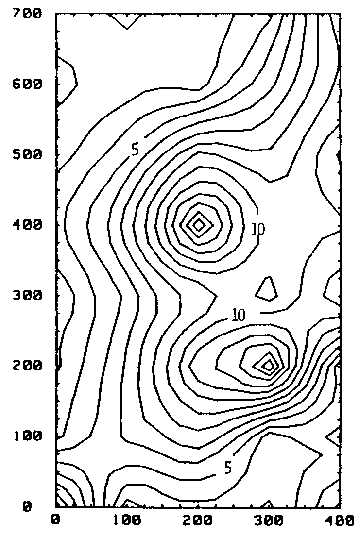
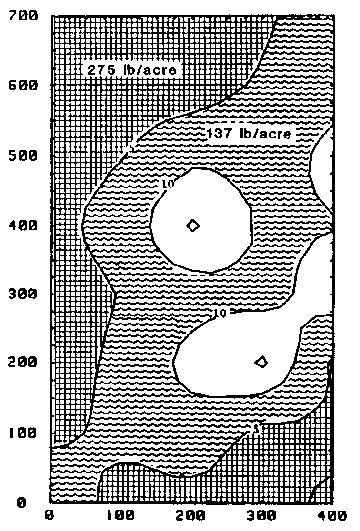
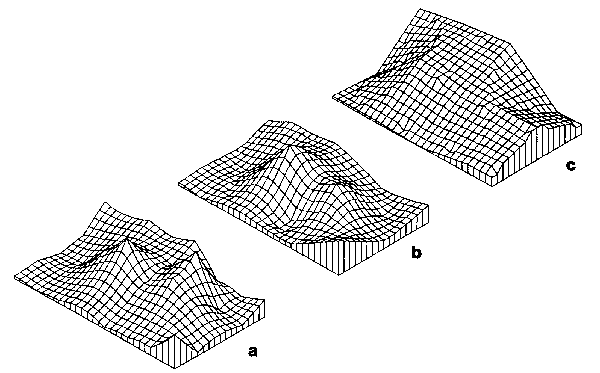