Maintaining Surface Residue on Summer Fallow
Chapter 3-Residue Management, No. 11, Fall 1988
Roger Veseth
Water is usually the major yield-limiting factor in dryland crop production. Seldom is rainfall sufficient or adequately distributed during the growing season for optimum yield. Consequently, it is necessary to have stored soil water available to supplement inadequate growing season precipitation. In much of the Pacific Northwest, stored soil water is especially important since only about 30 to 35 percent of the annual precipitation occurs during the growing season. The remaining 65 to 70 percent occurs the fall and winter from October 1 through March 31.
In low precipitation areas, a year of fallow in the rotation is often needed to increase the amount of plant available water, provided there is sufficient soil water storage capacity. Even though efficiency in storing available precipitation during fallow is typically about 40 percent in this region, the additional soil water greatly increases the chances of timely seeding and a successful crop. However, soil losses by wind and water erosion have been severe under conventional tillage on ”black” fallow. The erosion is particularly a problem during the winter after the fallow field has been seeded to winter cereals. Management practices which maintain more surface residue through the fallow season and after planting winter cereals can effectively reduce soil erosion. Higher surface residue levels also help maintain or improve water storage efficiency for optimizing yield potential.
Research Focus
The development of management strategies to improve water and soil conservation has been a major focus of the STEEP (Solutions To Environmental and Economic Problems) research program. Since 1975, this conservation farming research program has involved more than 100 USDA-Agricultural Research Service (ARS) and university scientists in Idaho, Oregon and Washington.
More than 20 STEEP scientists have been involved in some aspect of research on fallow management. One of the scientists involved in fallow water storage research is Robert Papendick, USDA-ARS soil scientist at Pullman, WA. Papendick is also the research leader for the ARS Dryland Soil and Water Conservation Research Unit at Pullman and serves as co-leader of STEEP.
Steps in Water Conservation
Papendick points out that there are three main objectives to conserving water: (1) holding the precipitation where it falls, (2) infiltrating the water into the soil and (3) minimizing evaporation losses of stored soil water, A fourth objective in soil water conservation for the dryer, summer fallow areas is to maintain adequate water in the seed zone for crop establishment.
Although water losses from runoff and deep percolation can be highly significant in some years and locations in the crop-fallow areas, losses normally comprise a relatively small percentage of the total precipitation. Where these losses are a problem, yield potential can be dramatically reduced. Transpiration losses of water by weeds can also be significant, but weeds can usually be controlled with tillage or herbicides. Evaporation usually accounts for the bulk of the water loss during fallow. Evaporation is also generally the most difficult water-loss process to control.
Evaporation Processes
Papendick explains that there are two main stages in the soil-water evaporation process. The first or constant-rate stage occurs when the soil surface is wet. The evaporation rate is at a maximum and is controlled by the evaporative demand of the atmosphere and soil surface conditions. Surface residue level, roughness and other surface conditions can modify the influence of atmospheric conditions by reflecting heat and slowing air movement near the soil surface.
On a bare soil, the first stage evaporative rate can approach that from an open pan of water. Water moves up to the soil surface as a liquid by capillary flow (similar to how water moves up in a sponge) and the rate of movement keeps pace with the evaporative loss. As drying continues, the rate of water movement through the soil eventually begins to lag behind the potential evaporative rate.
The second or declining-rate stage begins as the soil surface becomes dry, This stage is characterized by a rapid decline in the rate of water loss. Upward movement of liquid water by capillary flow eventually stops, and water loss occurs at a much slower rate by water vapor flow, As the thickness of the dry surface soil increases, the influence of atmospheric conditions on soil water evaporation decreases.
Factors Affecting Soil Water Storage Potential
Papendick lists several factors that affect water storage efficiency during fallow in the Northwest. These include precipitation and temperature patterns, weed control, soil characteristics, crop residue amounts, and tillage and residue management.
Precipitation and Temperature Patterns
Precipitation form (snow or rain), rainfall intensity and time of year during which the rainfall occurs are often just as important to conservation as the amount that falls. Snow is generally stored more efficiently than rain except when the soil is frozen. In most of the Inland Pacific Northwest, the period which has the greatest potential for increasing soil water storage is from October 1 to March 31, when about 65 to 70 percent of the annual precipitation falls and evaporative potential is relatively low. During the hot, dry summers, the focus of summer fallow management is on reducing evaporative losses, with little potential for increasing soil water content.
Typically, there is a net loss of water over the summer. In southeastern Idaho, where annual precipitation is distributed relatively uniformly through the months of the year, reducing evaporation losses during fallow is still very important because of the low precipitation levels.
Weed Control
Although water loss to weeds has been a very significant problem in the past, modem herbicides and tillage equipment combinations can effectively minimize weed growth throughout the entire fallow season. Papendick stresses that early and continuous weed control is important since large weeds and high weed populations can remove significant amounts of soil water. Minimizing weed and volunteer growth also appears to be important for reducing the potential for Rhizoctonia root rot and other soilborne diseases.
Soil Type
Soil type can influence water storage and retention during fallow through both soil water-holding characteristics and soil water conductivity properties. Sandy soils have a lower storage capacity than silts and clays but have higher infiltration rates. During extended dry periods with high evaporation potential, sands loose their liquid water capillary continuity to the surface more rapidly upon drying than do finer textured soils. For this reason, sandy soils are commonly called “self mulching. ” On finer textured soils, a tillage mulch is often more important than on sandy soils to break the liquid capillary continuity to the surface in order to effectively control evaporation during extended dry periods. Consequently, different management strategies for water conservation are often needed for different soil types.
Influence of Surface Residue
Surface residue effectively reduces runoff by slowing surface water movement downslope, allowing more time for infiltration into the soil. Standing stubble traps snow uniformly over the field, reducing drifting. Both the residue and the snow cover reduce soil freezing, helping to maintain water infiltration into the soil. Crop stubble also can help prevent freezing of the soil surface during late winter diurnal (night-day) freeze-thaw cycles by reducing heat loss from the soil during the night, With a bare soil surface, water moves upward to the frost zone at night. During daytime thawing, this water can be lost by evaporation. Significant water loss can occur over repeated freeze-thaw cycles, Surface residue helps reduce this evaporative loss by reducing the frequency and depth of soil freezing.
A residue mulch reduces soil water evaporation by insulating the soil from the sun’s heat and by slowing air movement over the soil surface, thus reducing the transfer of water vapor from the soil to the air. Papendick points out that surface residue reduces evaporation most during the cool, rainy season when the soil surface is wet and intervals between rains are relatively short. Slowing the evaporation rate under these conditions allows more time for water infiltration and storage.
Research by Papendick near Lind, WA, a 10-inch annual precipitation zone, illustrates the benefit of surface residue in water storage overwinter (Table 1). Storage efficiency with standing wheat stubble was 18 percent higher than with the bare soil. All of the summer precipitation was lost on all the treatments along with some of the water stored over winter. Net over summer water loss was higher with residue than without, Still, since the soil water content in March was higher with surface residue than without, September water storage amounts were still higher with surface residue.
Table 1. Effect of winter wheat residue level on fallow soil water storage near Lind. WA. 1974-75 (Papendick. USDA-ARS).
Residue Treatment (lb/acre) | Soil Storage of Precipitation | ||
---|---|---|---|
Sept-March 1 | |||
Storage (inches) | Efficiency (%) | March-Sept. Net Loss by September |
|
0 (burned) | 3.2 | 48 | 0.3 |
4,500 (original) | 4.3 | 66 | 0.6 |
10,000 (added) | 5.3 | 81 | 0.7 |
1 September-March precipitation was 6.5 inches. |
Several similar studies comparing overwinter soil water storage between standing wheat stubble and a bare surface (residue removed) have been conducted throughout the Inland Northwest and southeastern Idaho. Overwinter soil water storage with standing stubble generally ranged from about 15 to 45 percent higher than with a bare surface. Variations result from differences in precipitation zone, residue level, runoff, soil type, annual climatic fluctuations and many other factors.
Burying the residue by fall plowing can also result in a reduction of overwinter water storage when compared to standing stubble. Robert Ramig, USDA-ARS soil scientist at the Columbia Plateau Conservation Research Center near Pendleton, found that standing wheat stubble (about 9,000 lb/acre) stored about 20 percent (about 2 inches) more overwinter precipitation than with fall plowing. The study was conducted over 10 years in the Pendleton area in a 16 inch precipitation zone on Walla Walla silt loam soil.
Papendick points out that during the dry Northwest summers, when extended drying periods occur, total water loss from an untilled residue-covered soil can exceed the loss from a bare soil. This is because the residue allows the surface to remain moist for a longer period. Consequently, the first stage of evaporation on residue covered soil continues longer than on the bare soil. This is because the surface soil dries more slowly, and the slower second stage of evaporation does not begin as soon. As a result, no-till chemical fallow may not maintain adequate seed zone soil water over extended drying periods under some soil and environmental conditions in order to allow timely planting of winter cereals.
Both successes and failures in maintaining adequate seed zone soil water with no-till chemical fallow have been reported by researchers and growers. Soil type, precipitation level and distribution, residue amounts and position (standing vs. flat), and many other factors influence the success of no-till chemical fallow under the varied Northwest conditions, Papendick stresses that there is a need for more scientific research and grower experimentation to determine the applicability of no-till chemical fallow in the Northwest. An extensive computer modeling effort is underway in STEEP to identify the combination of conditions necessa~ for maintaining adequate seed zone water with no-till chemical fallow.
Effect of Tillage and Residue Management
Papendick points out that tillage and residue management during fallow should be approached from the standpoint of maximizing soil absorption of precipitation and minimizing evaporation losses during the dry periods. The number of tillage operations should be kept to a minimum to reduce soil erosion, water loss and production costs. Where runoff is a problem, particularly on frozen or compacted soil, a fall tillage, such as chiseling, can increase overwinter water storage. Without runoff problems, however, leaving the stubble standing overwinter usually results in water storage equal to, and in some cases greater than, storage after fall chiseling.
Papendick reemphasizes that in late spring, before the
hot, dry summer season, some tillage may be necessary to break the liquid capillary continuity between the seed zone and the surface soil. The dry tillage-mulch insulates the seed zone, minimizing water loss to slow vapor flow transfer, thus helping to maintain adequate seed zone water for germination.
There are three general approaches to tillage and residue management in summer fallow:
Conventional Tillage — Nearly all of the surface residue is buried with soil inversion-type, primary tillage implements, such as the disk or moldboard plow, and/or numerous secondary tillage operations.
Stubble Mulch — A significant portion of the previous crop residue is maintained on the surface through the use of subsurface soil mixing implements such as the chisel or sweep for the primary tillage, as well as the use of herbicides to delay and replace tillage operations.
Chemical Fallow – Volunteer and weeds are controlled exclusively with herbicides and the subsequent crop may or may not be direct-seeded without prior tillage.
Where tillage is used in summer fallow in the Pacific Northwest, the surface tillage-mulch has proven to be a fairly reliable system to maintain adequate seed zone soil water, enabling establishment of winter cereals in August or September. However, there appear to be some misconceptions about the role of surface crop residue in the mulch. Papendick and other STEEP researchers point out that both surface residue and tillage can improve soil water retention through the fallow season.
Cooperative research near Lind by John Harnmel, University of Idaho soil scientist, and Papendick points out the important benefit of both surface residue and tillage during 1975-76 fallow season, a “normal” precipitation year. Combinations of two residue treatments with and without tillage are compared in Fig. 1. The tillage treatment consisted of a twisted-shank chisel operation at 7-inch depth in May followed by two rodweedings at 4 to 5 inches depth to establish a dry tillage-mulch.
Residue treatments included total residue removal and the existing winter wheat stubble of approximately 4,500 pounds/acre. The residue level in September of the fallow season on the tillage treatment was about 1,500 pounds/acre (62 % surface cover). Weed growth was controlled on all treatments with a non-selective herbicide in order to isolate the effects of tillage and residue on soil water storage. The study was conducted on a deep, Ritzville silt loam soil, which is a common soil in crop-fallow areas of the Inland Northwest.
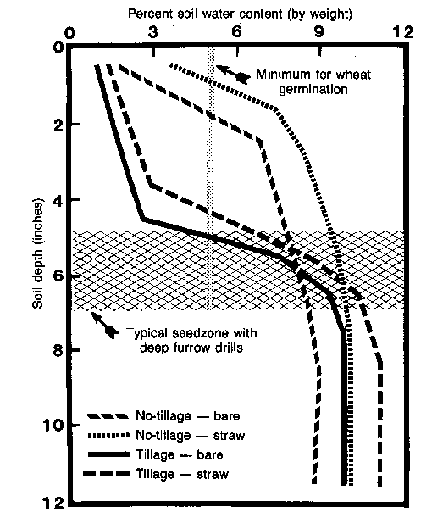
All treatments maintained adequate water content for wheat germination in the common seed zone depth for deep furrow drills under tilled conditions. Tilling the bare surface treatment increased soil water retention below about 6 inches compared to the bare no-till treatment. With surface residue but without tillage, water content increased throughout the profile when compared to bare no-till treatment. The combination of both tillage and surface residue maintained the highest soil water content below about 6 inches.
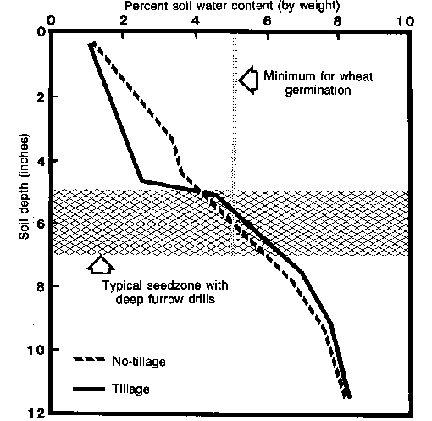
Hammel and Papendick also conducted fallow research during the same crop year on a fine sandy loam soil in the Horse Heaven Hills area near Richland, WA, in an 8-inch annual precipitation zone. They compared no-till chemical fallow to a tillage treatment which consisted of a sweep operation in March followed by two rodweedings (Fig. 2). The tillage treatment left about 1,000 pounds/acre surface residue (48 % surface cover) by the August planting, about half the original residue. The researchers found little difference in seed zone water between the two treatments. They attributed the lack of difference to the low evaporative loss from the “self mulching” sandy soils and to the initial low residue level.
Research by Ramig near Pendleton further shows that growers can effectively use both surface residue and a tillage mulch in maintaining adequate soil water storage. He conducted a fallow management study with six different tillage systems over five fallow-wheat cycles on a Ritzville silt loam soil. Each cycle extended for 18 months from September 1 after harvest, through the fallow season to March 1 in the next wheat crop year. No-till chemical fallow was not included in the study. Reduced tillage systems, which maintained 30 to 40 percent surface residue cover through winter wheat seeding, resulted in yields and stored soil water equal to or greater than with 5 to 30 percent surface residue under more intensive tillage systems.
Summary
The researchers conclude that by maintaining a portion of the crop residue on the soil surface through the fallow season and after seeding a winter cereal with conservation tillage, producers can reduce soil water losses compared to “black” conventional fallow. Historically, a dry tillage-mulch during the summer of the fallow season has helped maintain adequate seed zone water for timely fall seeding. The researchers emphasize that producers can retain more surface residue as part of the tillage mulch and still maintain or possibly improve seed zone water content, No-till chemical fallow has been successful in some areas and years in the region, but they stress that additional research is needed to help determine where and under what conditions it is most feasible.